This is a transcription of an article detailing the construction of the original Forth Road Bridge, now known to all as Kincardine Bridge. The article was first published in 1937, just a year after the bridge opened, and offers a fascinating contemporary insight in to the building process.
This version of the article has been created by obtaining a copy of the original magazine and using Optical Character Recognition (OCR) to generate the text. The images have been scanned at high resolution and are reproduced here at lower resolution to aid downloading. The layout has been changed from the original article in order to assist with displaying it on this website. If you would like to see the article in its original format you can find it at the wonderful Wonders of World Engineering website.
Our thanks to Will Anderson for purchasing the magazine and scanning the relevant sections for us. We are happy for anyone to use this information for their own personal research and ask that you contact us to let us know, as we are always pleased to hear from those who have enjoyed our work.
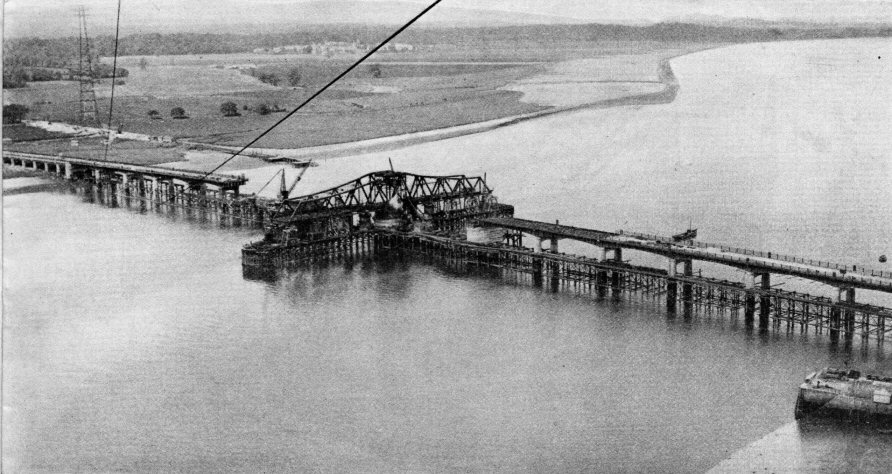
TWENTY-SEVEN SPANS and an approach viaduct, 265 feet long, make up the total length (2,696 feet) of Kincardine Bridge across the Firth of Forth. A temporary structure built from either bank on timber piles was provided to carry a double line of railway track for gantries, wagons and cranes during the building of the bridge.
LINKING THE WORLD’S HIGHWAYS
EUROPE’S LONGEST SWING BRIDGE
Across the Firth of Forth at Kincardine is a magnificent road bridge whose central span, 364 feet long, is pivoted on a central pier. This span is opened by electric controls to allow the passage of shipping.
For many years ferries have linked the north and south shores of the Firth of Forth, but most of the road traffic for central Scotland has had to make the crossing of the Forth at Stirling. In 1922 the average number of vehicles crossing Stirling Bridge in an hour was 150, but in 1935 this figure had risen to 400.
To relieve the congestion, the County Councils of Fife, Stirling and Clackmannan decided to build a new road bridge across the Forth. The site finally chosen was at Kincardine, below Alloa. Kincardine Bridge was designed by Sir Alexander Gibb and Partners, of London, and built by the Cleveland Bridge and Engineering Company, Ltd., of Darlington. The structure has a total length of 2,696 feet. At the northern or Kincardine end of the bridge the first three steel spans, each measuring 62 ft. 6 in., are built on a curve over the track of the London and North Eastern Railway. The curve was necessary to make a junction with a special approach road avoiding the streets of the town. The roadway on the curve is banked and at this end of the bridge is 15 feet higher than at the southern end.
The next seven spans, also of steel, are each 100 feet long and their design is of particular interest. The supporting system adopted is that of the cantilever – well in keeping with bridge practice on the Firth of Forth. Every alternate 100-feet span is made up of a central section, 50 feet long, which rests at either end on girders, 25 feet in length, projecting from adjacent spans. This method of construction permitted the use of the arched girders that are a pleasing and characteristic feature of the bridge. Each span consists of six arched girders which support a roadway 30 feet wide. The roadway is flanked by two 5-feet footways, which are carried on curved brackets cantilevered out from the bridge sides. The bridge piers comprise tapered cylinders of reinforced concrete, arranged in pairs which are joined by curved portal beams carrying the span girders.
In midstream is the magnificent central span. At the touch of an electric control wheel this can be swung on its supporting pier, leaving a clear passage of 150 feet on either side for shipping. This swing span, weighing 1,600 tons, is 364 feet long and its girder work towers 80 feet above high-water level. When closed, the swing span provides 30 feet of headroom above high water.
South of the swing span is another series of seven 100-feet steel spans. Next are nine 50-feet spans of reinforced concrete. A viaduct, also of reinforced concrete, provides the link with the southern bank of the river. This viaduct is 265 feet long, and sweeps slightly downward in a reverse curve to meet the lower level of the south approach. Apart from this reverse curve, however, the bridge is upswept from either end towards the centre span, giving it a graceful appearance when viewed from either shore.
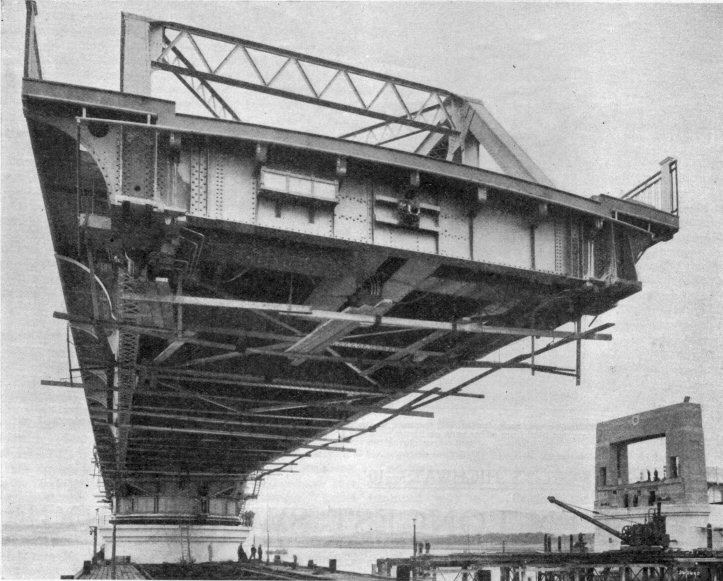
ONE END OF THE SWING SPAN of Kincardine Bridge contains the apparatus for securing the bridge in a closed position in perfect alignment. Just below the level of the roadway a box, 5 feet long, contains photoelectric cells. The bolt in the centre and the wedges at the sides can be driven only when the photoelectric apparatus is in alinement (sic) with its counterpart on the end pier of the fixed part of the bridge.
In 1890 borings were made in the river bed at Kincardine during the survey for a railway bridge proposed by the former Caledonian Railway (now L.M.S.). This bridge was not built, but the borings later proved of value to the engineers of Kincardine Bridge. Solid rock was available for foundations on the north side of the river, but the borings indicated a fault in midstream, with rock at too great a depth to be of any value to the builders. It was found that below the layer of mud on the southern bank there existed a belt of firm gravel at a depth of about 50 feet. The whole of the southern section of the bridge thus rests on reinforced concrete piles driven deep into the gravel.
The setting out of the site was accomplished from two positions, 2,000 feet apart, on the centre line of the bridge. The distance was determined by triangulation from a base line established on the L.N.E.R. embankment on the north bank of the river. This setting out was necessary to permit simultaneous building from either end. When, later, the staging had been completed on both sides, up to the central gap for the swing span, the opening was found to be one inch too wide. This small error was easily rectified on the midstream end piers.
With the position of Kincardine Bridge duly determined, the first task was the building of a temporary structure from which the main work could be carried out without delay. The temporary bridge had to be built substantially to serve for a period of three years, during which time its two sections, each a quarter of a mile long, had to carry a double line of standard-gauge railway track for the safe conduct of gantries, wagons and cranes across the fast-flowing Forth.
The bridge was built out from either bank on timber piles, a foot square in section, driven deep down to the solid rock. The pile heads were joined together by massive timbers and heavy steel joists carried the track. The north bank bridge staging carried two lines of railway track. One track ran along the centre line of the main bridge site, the other being arranged parallel to it, on the downstream side. This arrangement was adopted for the building of the north piers, as most of them were built with the help of caissons. Excavated material was loaded into wagons on the centre line track and the cranes ran along the outer or downstream set of metals.
For the southern piers of the main bridge, built entirely all piles, other methods were used. The two tracks surmounting the temporary staging were both situated outside the bridge site at a distance of 62 feet between centres. Each track carried the ends of a travelling gantry on which was erected a piledriver capable of working vertically or at an angle. It was not practicable to use wooden piles for the southern section of the temporary bridge, which was built on a raft of wooden sleepers laid on top of the coarse grass that covered the saltings of the south bank.
The building of the northern section of the temporary timber bridge was completed in August 1934 after six months’ work. The southern section was begun in July 1934 and was nearly completed by February 1935 when a steamer, inward bound for Alloa, crashed into the staging early one morning. Considerable damage was done to the timbering and the crane. This unfortunate accident caused much delay, but finally the southern section was completed to within 150 feet of the staging from the north bank of the river. The whole of the staging was illuminated by electric lamps for the guidance of shipping at night time, and the gap for shipping was left between Piers 11 and 12.
The permanent timber jetty in midstream, which protects the swing span when it is opened for shipping, was completed by the autumn of 1935. This structure comprises four rows of 14-in. piles, 50 feet long and driven 10 feet into the river bed. The ends of the jetty are rounded and strongly braced to withstand any collision. The timber used was Canadian Douglas fir and the logs were “incised” before leaving Canada. These incisions were 3/4-in. deep, spaced 1 in. apart with 2 in. between the rows, and their object was to assist the penetration of the creosote used for preserving purposes.
The building of the concrete piers for the main bridge, with its half-mile of girderwork, occasioned not a little anxiety to the engineers in charge. With modification of design, however, and with the adoption of special methods of construction, all difficulties were finally overcome.
“Skin” of Concrete
Nearly all the concrete piers on the northern section were founded on solid rock. The exceptions were the north abutment and the first two piers carrying the three 62 ft. 6 in. end spans. These structures were built on piles driven down to the solid rock through soil that had been reclaimed from the river during the building of the railway embankment along the north bank. Pier 3 was the first to be built on the solid rock below the river bed. For the excavation of the foundations two steel cylinders were lowered by a crane from the temporary bridge to the mud of the river bed on either side.
The cylinders, having a diameter of 15 ft. 6 in., were.built of 1/4-in. steel plate stiffened by angles, and they comprised a number of sections bolted together. Timber guides were provided to maintain the cylinders in an upright position. As the material was removed from within by a grab and crane the steel shell sank down to the rock. The final excavation was done at low water by men in the cylinder. During excavation for the foundations of this pier a seam of good quality coal, 18 in. thick, was pierced through. With its base firmly set into the rock each cylinder was filled with concrete to a line about midway between Jow and high water marks. Tapered steel shuttering was then built on top of the concrete for the construction of the pier columns. These columns consist of a “skin” of concrete, 9 in. thick, heavily reinforced by steel rods.
As the outer ring of high grade concrete rose, the interior was filled in with special concrete and the steel shuttering was removed for use on other columns. The surplus sections of the foundation caissons were also removed when they had served their purpose as cofferdams. The twin pier columns were finally united at the top by a portal beam, also of reinforced concrete, to support the girders of the bridge.
Piers 4 and 5 were built in a similar manner. At Pier 5, still farther out in the river, the water found its way into the cylinders and it was necessary to employ a diver to seal the gaps at the base with bags of concrete. Similar difficulty was experienced with Pier 6, and it was decided that the time had arrived for the use of sealed cylinders and compressed air.
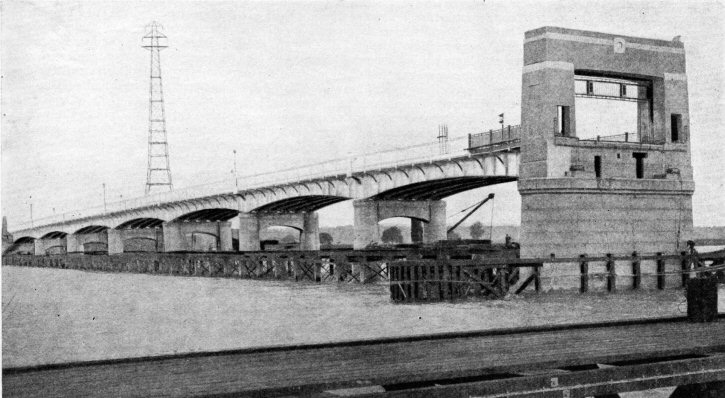
A MASSIVE CONCRETE PORTAL is built above the pier abutting on the swing span. A portcullis, weighing nearly four tons, is raised and lowered electrically inside the portal to open and close the road to traffic. Seven spans of 100 feet carry the road up to the portal.
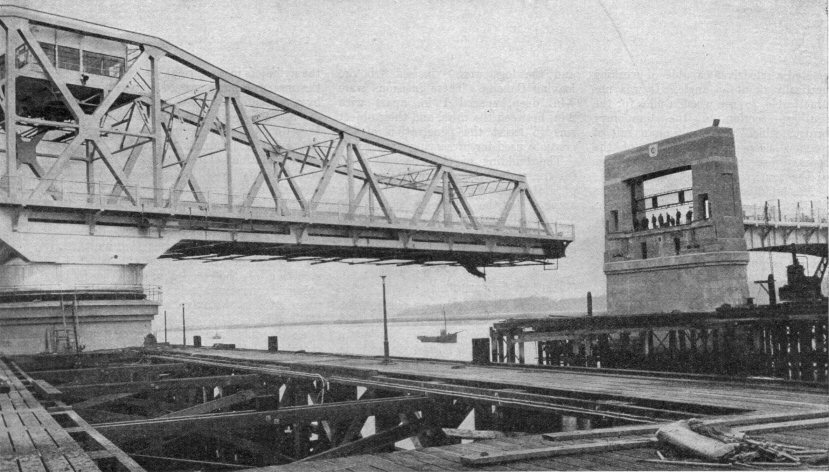
THE SUPERSTRUCTURE of the swing span of Kincardine Bridge comprises two enormous Warren girders. The roadway is carried on cross girders 5 feet deep. The weight at the centre is carried on two longitudinal girders 8 ft. 4 in. deep and on two transverse girders 11 feet deep.
The steel cylinders used for the first few piers were converted for use as compressed air chambers. The lower end of each was fitted with a coneshaped plate to form the roof of the working chamber. Leading up from this chamber two shafts, joined together longitudinally, were arranged. One shaft, smaller than the other, accommodated a steel ladder; the larger shaft permitted the hoisting of buckets of spoil by the crane overhead. At the top of the twin shafts were arranged the air locks for the men and material. Compressed air was introduced into the shafts and between these and the outer casing water was pumped in to weight down the caisson.
It was possible with these caissons to work on the pier foundations in all conditions of the tide. They were first used at Pier 9, last of the “standard” piers on the northern section of the bridge, and work progressed shorewards to Pier 6. This course was adopted so that the travelling crane could use the central track. On completion of two adjacent columns this track was obstructed, for crane use, by the portal beams. With the removal of material from within the cylinders they sank lower and lower into the river bed, and water was excluded by air at a pressure of 18 lb. per square inch.
When the cutting edge of a cylinder had entered the bedrock excavation to a depth of about 3 feet, a plug of concrete was placed inside to form a foundation. This operation was carried out under air pressure and, to prevent the passage of air or water through the concrete during setting, pipes were run from beneath the lower edge of the cylinder to a point above the top of the plug. After the concrcte had set the pipes were sealed and the water ballast was replaced by solid material. The cone plate shafts and air locks were removed for use in another cylinder, and work proceeded on the concrete column within the plugged, open-topped caisson.
The corresponding piers on the opposite side of the river were built on piles of concrete reinforced by steel rods. The piles were 18 in. square, between 55 feet and 65 feet long, and weighed 9 tons. The reinforcing comprised four long steel rods joined with transverse links at close intervals; round the rods the concrete was cast in shuttering. A special pile-casting yard was laid out on the south bank of the river. The piledriver comprised a carriage 90 feet long, and the piles, protected by a steel helmet with a timber cap, were driven by a steam hammer weighing 5 tons. Six hammer blows were allowed for every inch of drive and the drop of hammer head was limited between the 3 ft. 9 in. and 4 ft. 3 in., according to the length of the pile. The weight on each pier was 1,250 tons, spread over eighteen piles. The number of reinforced concrete piles used was 484.
The building of the pile-supported piers began with the lowering of a cylinder, 14 ft. 6 in. in diameter, into the river bed for a distance of ten feet. Similar methods were used to those adopted for the piers close to the north bank of the river. This procedure permitted pile-driving within the caisson. After the driving of the piles a concrete plug was deposited, under water, in the bottom of the cylinder. When this concrete had set, the cylinder was pumped dry, leaving about 18 feet of each pile projecting through the plug. The cylinder was then filled up with concrete to within about three feet of low-water level and the ends of the piles still projecting were built into the base of the tapered column rising from the cylindrical concrete base. The steel cofferdam was in each instance finally removed, and every pair of columns was provided with a reinforced concrete portal as in the north piers.
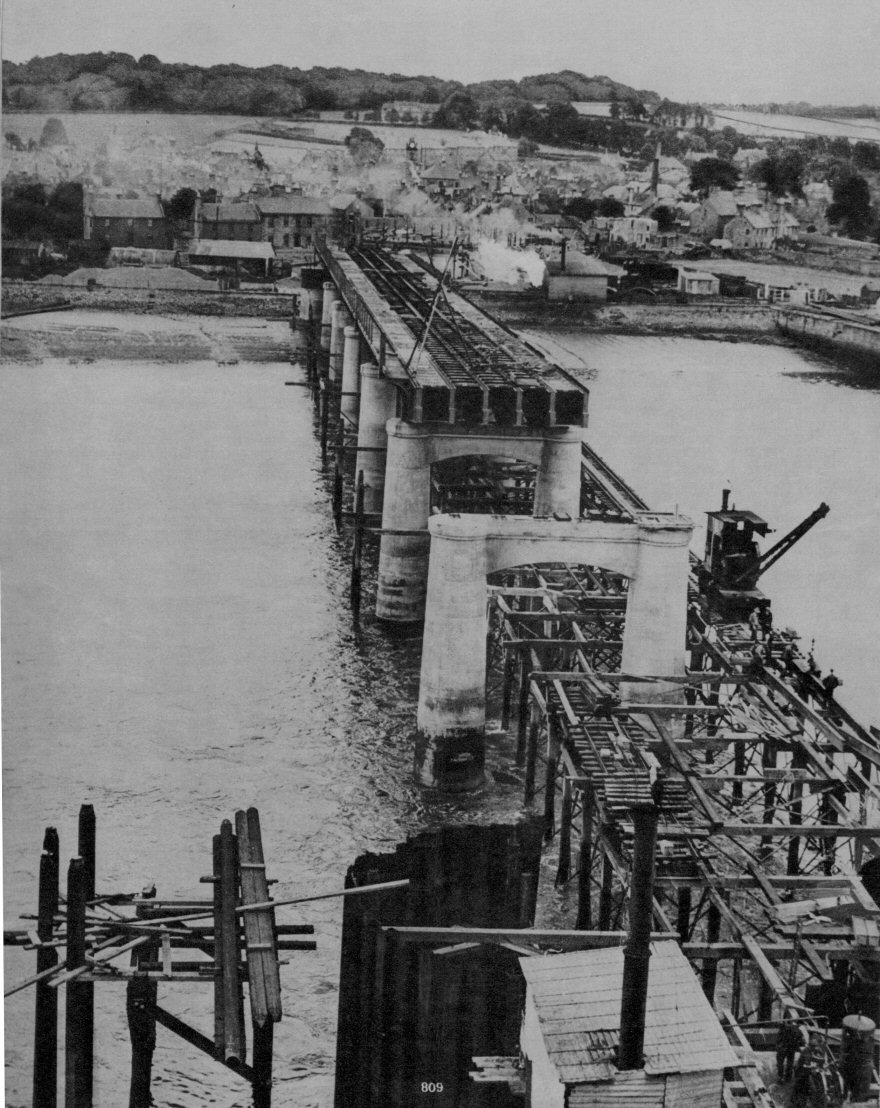
GIRDERS OF THE 100-FEET SPANS were placed in position from the temporary bridge shown on the right of the photograph. Two girders were handled at a time. Those on the upstream side were rolled by jacks across steel balls in V-shaped tracks along the tops of the piers.
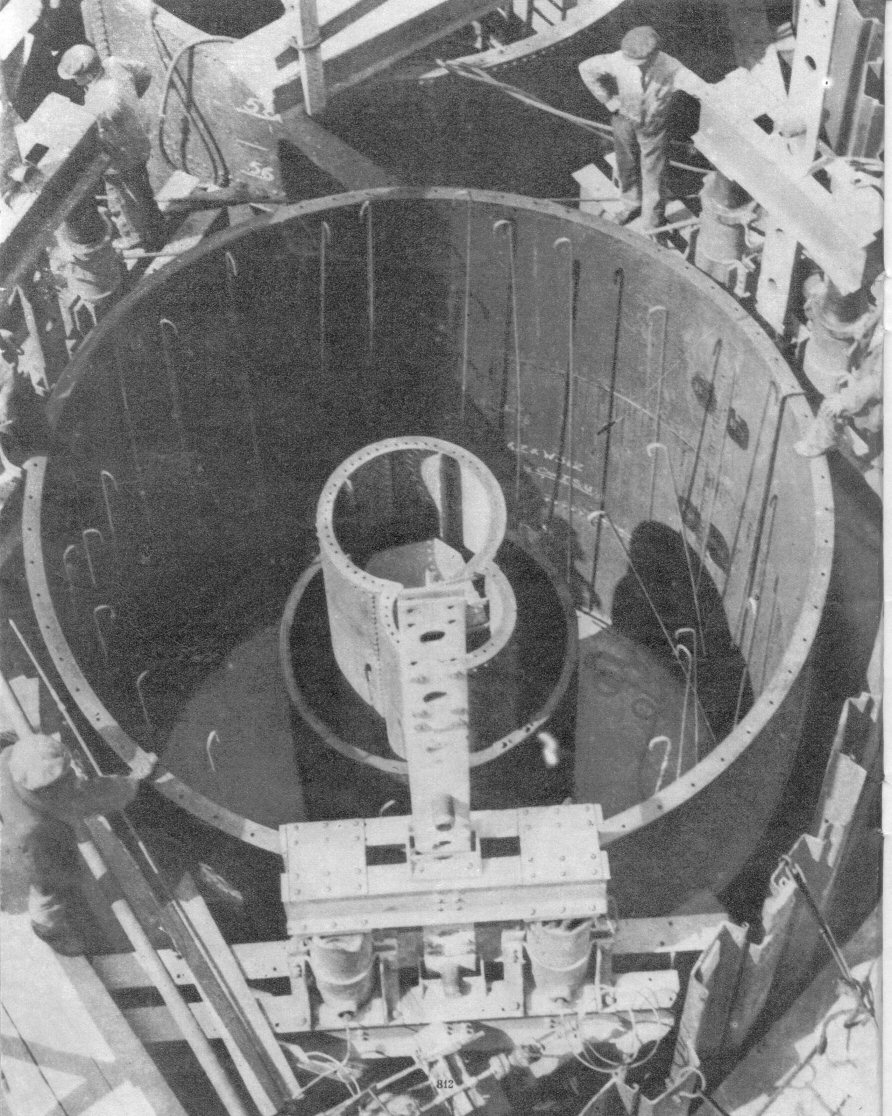
FOUNDATIONS FOR THE CENTRAL PIER of Kincardine Bridge were made by sinking to rock level, under air pressure, six cylinders with a diameter of 14 ft. 6 in. The cylinders were lowered by hydraulic jacks. Concrete was placed between the cylinder walls and the circular shuttering round the air shaft. As the concreting proceeded the jacks were adjusted and the cylinders sank slowly to their foundations.
The piers for the 50-feet reinforced concrete spans of the bridge were built in a manner similar to that used for the l00-feet spans. Cylinders were unnecessary, however, as the saltings on which the piers were built are covered only by spring tides. The viaduct at the south end of the bridge was built on 18-in. piles, 65 feet long, driven 50 feet into the ground. The piles are spaced at 10-feet centres longitudinally and at 15-feet centres transversely; they support a reinforced concrete roadway 10 in. thick. Concrete footpaths, 8 in. thick, are cantilevered out from the sides.
The most important pier in the whole bridge is undoubtedly Pier 11, the huge mass of concrete on which swings the great central span. The original design for this pier provided for a huge hollow drum of concrete 42 feet in diameter, with walls 5 feet thick, founded upon the rock below the river bed. For the carrying out of this work a steel cofferdam 50 feet in diameter was lowered through 12 feet of sand and gravel to bedrock. In December 1934 the pumping of water from the interior of the cofferdam was begun, and at one time the pumps were handling 6,500 gallons a minute. Several minor inrushes of river water took place despite the thick layer of sand outside the dam. At high water on January 9, 1935, there occurred a violent onslaught by the river and some 20 feet of sheet steel were burst inwards, completely flooding the great cylinder.
It was decided that, rather than attempt a resealing of the cofferdam, the design of the pier would have to be modified, and six cylinders 14 ft. 6 in. in diameter were sunk under air pressure to rock level. The cylinders were equally spaced out on a diameter of 33 feet and were lowered by the use of hydraulic jacks from a temporary staging. The concrete of the column was placed between the cylinder wall and the circular shuttering surrounding the central air shaft. As the concreting proceeded, the jacks were adjusted and the cylinders sank slowly to their foundations. When built to a level just below low water the tops of the six concrete columns were united by a huge slab of heavily reinforced concrete, 4 feet deep, and the steel cylinder sections were removed. Above the concrete slab the enormous drum, 42 feet in diameter, was built as originally designed.
The top of the pier consists of two steel lattice girders 6 ft. 6 in. deep, arranged at right angles. At the junctions of the girders are eight U-bolts that secure the central pivot of the swing span. The girders are totally enclosed in concrete and the sloping roof of the pier is of reinforced concrete from 27 in. to 19 in. thick. The interior of the pier is ventilated by gratings, and a manhole in the roof provides access down an iron ladder. The total weight on the foundations of this pier is 4,200 tons.
The experience gained in the building of the central pier proved of great value in the erection of the neighbouring north pier, Pier 10, and similar methods were adopted. Both piers are more substantial than the remainder because they have to support, in addition to the roadway, the ends of the swing span when it is in the road position, and the vertically sliding gates that close the bridge ends. These piers also afford protection against passing shipping.
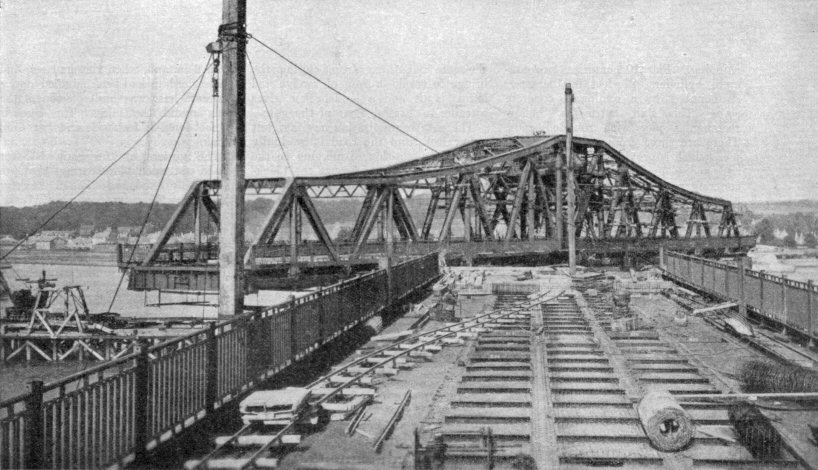
REINFORCED CONCRETE ROADWAY, with its top layer of asphalt, was built on steel buckle plating between the top, of the girders. The roadway is 30 feet wide. Footpaths, 5 feet wide, were cantilevered out from the outer girders.
Pier 10 comprises two concrete cylinders, 21 feet in diameter, surmounted by a reinforced concrete slab. This slab carries a hollow rectangular pier with rounded ends. There are three cross walls inside the pier, which is provided with ventilators and access manholes. The reinforced concrete roof is 4 feet thick and this carries the ends of the 100-feet girders and the swing span supports. Above the roadway is a massive concrete portal, providing an opening 18 ft. 9 in. high and 32 feet wide. The portal bears the arms of Fife, Stirling and Clackmannan carved in Portland stone. A gate, weighing nearly four tons, slides portcullis-fashion within the portal and is raised or lowered electrically. As the gate begins to drop, closing the bridge to traffic, a row of twelve red lamps is lit and a loudspeaker gives warning to “stand clear of the gate.”
The gate begins its journey fast but a few feet from the road it gradually creeps into position, displaying to the traveller in letters of bronze the Clackmannan motto “Look aboot ye” advice well worth following with an engineering marvel before and the glorious Scottish scenery all round.
Pier 12, on the southern side of the central span, is built on reinforced concrete piles. In this instance it was found possible to use a steel cofferdam within which the piles were driven. The whole area of the cofferdam was plugged with concrete to a depth of 3 ft. 6 in. and, when this had set, and the cofferdam had been pumped dry, a concrete base was built on top of the plug round the piles. The base is 65 feet long, 27 feet wide and 7 ft. 6 in. thick.
The girders of the main 100-feet spans rest upon pier bearings that are alternately fixed and sliding. Each of the sliding bearings comprises a castiron bedplate 3 in. thick with a projecting tongue keyed into the concrete. This surface is faced with a phosphor bronze plate. On the underside of the girder is attached a similar plate of steel to form the upper bearing surface. Alternate 100-feet girders are linked by 50-feet suspension girders, thus forming a system of cantilevers.
The steel was hauled from Kincardine Station, adjoining the north abutment, along the downstream track of the temporary bridge. The components of two contiguous girders, with their cantilever ends, were placed in position alongside one another on the downstream side of the bridge by two 5-tons locomotive cranes. These girders were then braced together and the whole mass of steel, weighing about 55 tons, was rolled by jacks to the upstream side of the bridge. This operation was carried out by the use of steel balls rolling in V-shaped tracks along the tops of the piers. Two more girders were then rolled into a central position and finally the remaining pair was placed on the downstream side to complete the series of six longitudinal girders between adjacent piers.
The reinforced concrete roadway, with its top layer of asphalt, was built on steel buckle plating between the tops of the girders. The cantilevered footways were provided with granite kerbs 8 in. high. Beneath the concrete paving ducts were arranged for cables and water pipes. The weight of steel used in the bridge amounted to 4,000 tons, and no fewer than 150,000 rivets were driven at the site.
The swing span at Kincardine, the longest in Europe, is a perfect combination of electrical and mechanical excellence. Despite its weight of 1,600 tons, the swing span is operated by electrical machinery at a trifling cost. This machinery performs the turning and operates the gates and hydraulicgear on a consumption of only 2’1 units for a swing through an angle of 900 and back again.
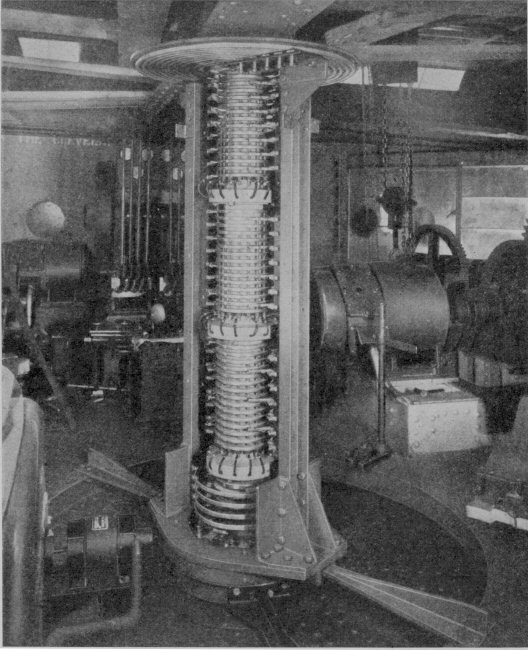
SLIP RING COLUMN in the machinery room ofthe swing span. The column is bolted to the fixed pivot round which the span rotates. Brush gear, rotating with the span, collects electric current from the rings, of which there are sixty. This arrangement of brushes and slip rings is generally adopted to supply electricity to rotating parts.
The superstructure comprises two enormous Warren girders and the floor carrying the roadway consists of cross girders 5 feet deep. The weight of the span is carried at the centre on two longitudinal girders 8 ft. 4 in. deep and on two transverse girders 11 feet deep. These girders rest on a drum, or circular girder, which is stiffened by eight radial girders that support the floor of the machinery room. This is situated between the girders and has for a roof the floor of the deck. Beneath the lower edge of the drum girder is secured a cast-steel tapered track which rests on sixty caststeel rollers. These rollers are bored through the centre to take a phosphorbronze sleeve, inside which is the outer end of a steel radial rod joined to a central pivot. There are thirty of these rods that prevent the rollers from being forced, in the manner of an orange pip between the fingers, in an outward direction by the combined action of the tapers. The rods serve also to support a huge circular cage that spaces out the rollers. The whole roller system with its cage resembles, on an immense scale, a roller thrust bearing as used in motorcar practice.
Turning Machinery
The rollers run on a lower track, also of cast steel, mounted on steel beams partly embedded in the concrete of the pier. This lower track is level to within a limit of two-thousandths of an inch and the rollers are machined to within a liinit of three-thousandths in taper and in diameter. Accuracy of this degree in such large components explains the remarkably small amount of power that is required to swing the huge span.
A cast-steel rack, to engage with a gear system leading into the machinery room, is bolted to the outer edge of the lower roller track and to this in turn is secured a circular guard plate to prevent the ingress of rain or snow to the rollers. A similar guard, projecting downwards, is attached to the drum girder above the rollers.
The setting out of the roller track was done in February 1936. The load on each roller is 62 tons and lubrication is by grease under pressure. The centre pivot is a steel casting, with a diameter of 7 ft. 6 in. at the base and a height of 7 feet. Round this pivot revolves an upper collar, lined with phosphor bronze, which carries the inner ends of the eight radial arms of the drum girder. A similar lower collar carries the tie rods from the rollers.
At either end of the swing span, beneath the centre of the roadway, is a steel locking bolt mounted on a rectangular shaft and operated hydraulically at a pressure of 1,000 lb. per square inch. The tapered bolt-heads drive into cast-steel bevelled sockets in the outer faces of Piers 10 and 12.
The great length and weight of the swing span cause a slight sag at the ends. To counteract this, when the bridge is set for road traffic, four hydraulic rams are placed under the girderwork, one at each corner. These rams are fitted with tapered wedges that are forced over rollers mounted on the end piers. A lift of about 2 in. is obtained at each roller under a pressure of 52 tons. These wedge rams work at a pressure of 3,000 lb. per square inch, but this figure can be exceeded by 50 per cent if necessary.
The turning machinery comprises two separate units, each consisting of a 50 horse-power direct-current motor geared to the rack on the pier. The pinions of the sets of gearing are used at opposite sides of the rack and the bridge can be swung completely round in either direction.
Direct current for the motors is obtained from a generating set which also supplies current for the electrical control devices. Alternating current for driving the generating set and for other purposes is supplied by cable from the Fife Electric Company. This cable goes down through Pier 10, along a trench in the river bed and up inside the central pier to a junction box in the roof. From there the cable is taken up through the swing pivot to a fixed column, equipped with a number of slip rings, in the centre of the machinery room. Contact brushes that can revolve with the swing span take current from the slip rings, and are connected by cables to the electrical machinery and equipment.
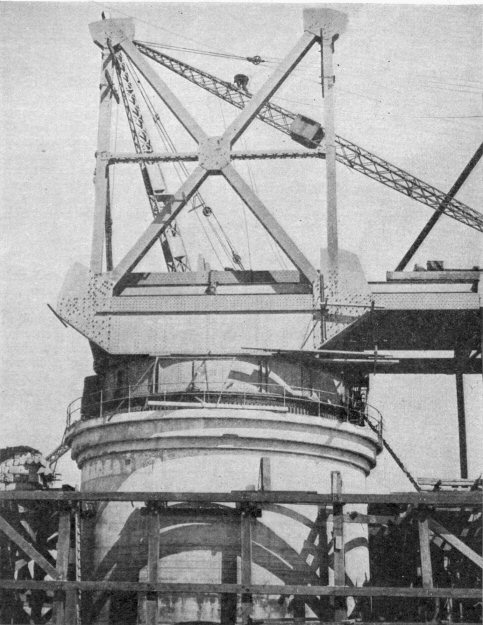
STEELWORK BEING ERECTED on the centre of the swing span. The deep girders rest on a drum, or circular girder. On the bottom edge of the drum girder is fixed a cast-steel track which rests on sixty cast-steel rollers.
Other machinery includes a 25 horsepower electric motor that drives a geared six-throw hydraulic pump, for the operation of the bolts and wedges. Oil instead of water is used in the rams, to obviate any risk of freezing in winter. A diesel generator of 120 brake horse-power is provided as a stand-by in the event of failure of the electrical Grid, the power lines of which span Kincardine Bridge diagonally on two immense towers.
The machinery in the room below the roadway is controlled from a spacious cabin built 30 feet above the road on a bridge between the tops of the girders of the span. Access is by a steel ladder.
The whole of the lighting and equipment of the swing span, including the signals and gates on the end piers, is controlled from a desk through a main switchboard of three sections.
When it is required to swing the bridge so as to permit the passage of a vessel, the control wheel pointer is turned in the required direction of swing to Stud 1. The generator set immediately starts up and a relay sets the direction of swing. The pointer turns farther to Stud 2 and the hydraulic pump motor starts on light load. At Stud 3, the bridge traffic lights change from green to amber, then to red; bells ring at each portal and pedestrian warning lamps show red. The assistant operator then descends to the roadway to ensure that the bridge is clear, a task in which he is assisted by loud speakers which are operated from the cabin.
How the Bridge is Swung
The pointer is next turned to Stud 4 and the safety gates, with their red lights, begin to close. The lower of two semaphores on the jetty drops and an amber light changes to red, indicating to the vessel that the bridge is about to swing. A siren on the cabin roof sounds for six seconds. At Stud 5 the four wedges are withdrawn simultaneously. With the pointer at Stud 6 the bolts are withdrawn simultaneously and the hydraulic pump motor stops. The pointer turns to Stud 7 and the bridge begins to swing. Automatically it accelerates for 10 seconds, then moves uniformly for 100 seconds and finally decelerates for 10 seconds. Ten seconds after the bridge has stopped, and not before, brakes are applied which hold the swing span in position along the jctty in midstream. At Stud 8, the upper semaphore arm at either end of the jetty is lowered, a second amber light shows, the siren sounds for two seconds and the vessel may then proceed through the opening. The same sequencc is reversed when the span is returned to the road position.
Of special interest are the means adopted to centre the span before the locking bolts and wedges are driven. This is accomplished automatically by the use of a photoelectric device, whose operation is described on pages 390 and 442. Kincardine-on-Forth Bridge cost £252,000 and was opened for traffic on October 29, 1936.
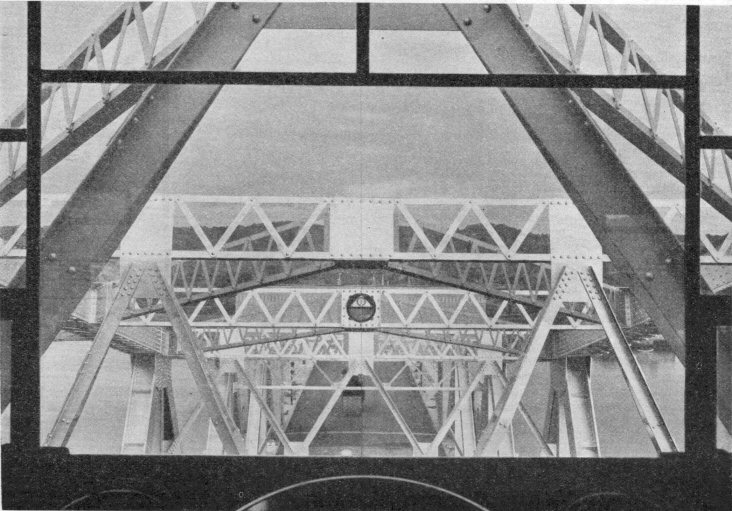
VISUAL ALINING (sic) EQUIPMENT as seen from the operator’s control desk. A vertical piano wire is fixed on the exact centre line of the bridge close to the window of the control cabin. This forms the backsight for an illuminated target on the abutment portals. The foresight is formed by a circular frame on the upper bracing of the swing span. This frame is glazed with transparent red and green glass screens. with a vertical white strip between. The light from the target shows through this strip only when the bridge is in perfect alinement (sic).
Pingback: Bridges to the Kingdom - Kilbride Cottage, Fife, Scotland